TREATING OMICRON BA.4 & BA.5 VIA HERBAL ANTIOXIDANT ASAFOETIDA: A DFT STUDY OF CARBON NANOCARRIER IN DRUG DELIVERY
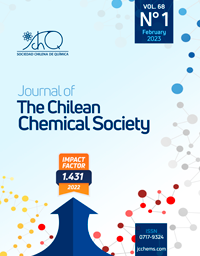
- Omicron subvariants BA.4 and BA.5, Asafoetida; Ferulic acid; CNT; drug delivery
Copyright (c) 2023 SChQ

This work is licensed under a Creative Commons Attribution-NonCommercial-ShareAlike 4.0 International License.
Abstract
Currently, the results of researches have exhibited that Omicron sub-lineages BA.4 and BA.5, evaluated to BA.1 and BA.2, deserted neutralization from sera of triple vaccinated particulars to a bigger extension. Therefore, a novel wave of Omicron virus has appeared driven by BA.4 & BA.5 subvariants. Nanocarriers contain carbon atoms with functional nanostructures, which not only help the improved mechanical properties but also indicate the bioactivities for regulating cell status. In this research, asafoetida as a medicinal plant can be applied in treatment for Omicron subvariants BA.4 and BA.5 through adsorbing of its effective compound of ferulic acid on the surface of (6,6) armchair single-walled carbon nanotube as the drug delivery model due to direct electron transfer principle which has been studied by density functional theory (DFT) methods.
On the other hand, it has been accomplished the B3LYP/6-311+G (2d,p) level of theory to evaluate the aptitude of SWCNT for adsorbing effective compound in asafoetida medicinal plant through nuclear magnetic resonance and thermodynamic parameters. In fact, the achieved results have represented that the feasibility of using (6,6) armchair SWCNT and ferulic acid becomes the norm in drug delivery system which has been attained by quantum calculations due to physico-chemical properties of NMR, IR and UV-VIS spectroscopy. Besides, the energy gap analysis of HOMO-LUMO has illustrated the charge distribution in the frontier molecular orbitals of ferulic acid in asafoetida drug through adsorption on the surface of (6,6) armchair carbon nanotube (CNT).
References
- Hansen, J. et al. Studies in humanized mice and convalescent humans yield a SARS-CoV-2 antibody cocktail. Science 2020, 369, 1010-1014. doi:10.1126/science.abd0827.
- Shahriari, S.; Monajjemi, M.; Mollaamin, F., Determination of proteins specification with SARS- COVID-19 based ligand designing, J. Chil. Chem. Soc. 2022, 67, 5468-5476. doi: 10.4067/S0717-97072022000205468.
- Zost, S. J. et al. Potently neutralizing and protective human antibodies against SARS-CoV-2. Nature 2020, 584, 443-449, doi: 10.1038/s41586-020-2548-6.
- Mollaamin, F. Function of anti-cov structure using inh [1-6]-tyr160-met161-his162 complex. Biointerface Res. Appl. Chem. 2021, 11, 14433-14450. doi:10.33263/BRIAC116.1443314450.
- Jones, B. E. et al. The neutralizing antibody, LY-CoV555, protects against SARS-CoV-2 infection in nonhuman primates. Sci Transl Med 2021, 13, doi:10.1126/scitranslmed.abf1906.
- Mollaamin, F.; Esmkhani, R.; Monajjemi, M. Mutations in novel covid-19 make it more dangerous: Prevention via scientific approaches. Biointerface Res. Appl. Chem. 2021, 11, 10546-10558. doi: 10.33263/BRIAC113.1054610558.
- Shi, R. et al. A human neutralizing antibody targets the receptor-binding site of SARS-CoV-2. Nature 2020, 584, 120-124, doi:10.1038/s41586-020-2381-y.
- Monajjemi, M.; Shahriari, S.; Mollaamin, F. Evaluation of coronavirus families & COVID-19 proteins: Molecular modeling study. Biointerface Res. Appl. Chem. 2020, 10, 6039-6057. doi:10.33263/BRIAC105.60396057.
- Cao, Y. et al. BA.2.12.1, BA.4 and BA.5 escape antibodies elicited by Omicron infection. bioRxiv, 2022, doi:10.1101/2022.04.30.489997.
- Mollaamin, F.; Monajjemi, M. Thermodynamic research on the inhibitors of coronavirus through drug delivery method. J. Chil. Chem. Soc. 2021, 66, 5195-5205. doi: 10.4067/S0717-97072021000205195.
- Khan, K. et al. Omicron sub-lineages BA.4/BA.5 escape BA.1 infection elicited neutralizing
- immunity. medRxiv, 2022, doi:10.1101/2022.04.29.22274477.
- Mahendra, P.; Bisht, S. Ferula asafoetida: Traditional uses and pharmacological activity. Pharmacogn Rev. 2012, 6, 141-146. doi:10.4103/0973-7847.99948.
- Mollaamin, F. Physicochemical investigation of anti-COVID19 drugs using several medicinal plants, J. Chil. Chem. Soc., 2022, 67(2), 5537-5546. doi: 10.4067/S0717-97072022000205537.
- Cannon, G.H.; Kaye, A.S. The Persian Contributions to the English Language: An Historical Dictionary. Otto Harrassowitz Verlag. 2001, ISBN 978-3-447-04503-2.
- Tiwari, G.; Tiwari, R.; Sriwastawa, B.; Bhati, L.; Pandey,S.; Pandey, P.; Bannerjee, S.K. Drug delivery systems: An updated review. International Journal of Pharmaceutical Investigation.2012, 2, 2-11. doi:10.4103/2230-973X.96920.
- Li, J.; Zeng, M.; Shan, H.; Tong, C. Microneedle Patches as Drug and Vaccine Delivery Platform. Current Medicinal Chemistry. 2017, 24, 2413-2422. doi:10.2174/0929867324666170526124053.
- Tekade, R.K. Basic fundamentals of drug delivery. 2018, ISBN 978-0-12-817910-9. OCLC 1078149382.
- Allen, T. M. Drug Delivery Systems: Entering the Mainstream. Science. 2004, 303, 1818-1822. doi:10.1126/science.1095833.
- Singh, A.P.; Biswas, A.; Shukla, A.; Maiti, P. Targeted therapy in chronic diseases using nanomaterial-based drug delivery vehicles. Signal Transduct Target Ther. 2019, 4, 33. doi:10.1038/s41392-019-0068-3.
- Cao, X.; Deng, W.; Fu, M. et al., Seventy-two-hour release formulation of the poorly soluble drug silybin based on porous silica nanoparticles: in vitro release kinetics and in vitro/in vivo correlations in beagle dogs. European Journal of Pharmaceutical Sciences, 2013, 48, 64-71. doi:10.1016/j.ejps.2012.10.012.
- Mollaamin, F.; Monajjemi, M. Harmonic Linear Combination and Normal Mode Analysis of Semiconductor Nanotubes Vibrations. J. Comput. Theor. Nanosci 2015, 12, 1030-1039. doi:10.1166/jctn.2015.3846.
- Zhang, L.; Xue, H.; Cao, Z.; Keefe, A.; Wang, J.; and Jiang, S. Multifunctional and degradable zwitterionic nanogels for targeted delivery, enhanced MR imaging, reduction-sensitive drug release, and renal clearance, Biomaterials, 2011, 32, 4604-4608. doi:10.1016/j.biomaterials.2011.02.064.
- Ghalandari, B.; Monajjemi, M.; Mollaamin, F. Theoretical Investigation of Carbon Nanotube Binding to DNA in View of Drug Delivery. J.Comput.Theor.Nanosci 2011, 8, 1212-1219. doi:10.1166/jctn.2011.1801.
- Monajjemi, M.; Honaparvar, B.; Khalili Hadad, B.; Ilkhani, A.; Mollaamin, F. Thermo-Chemical Investigation and NBO Analysis of Some anxileotic as Nano- Drugs. African journal of pharmacy and pharmacology 2010, 4, 521-529.
- Khaleghian, M.; Zahmatkesh, M.; Mollaamin, F.; Monajjemi, M. Investigation of Solvent Effects on Armchair Single-Walled Carbon Nanotubes: A QM/MD Study. Fuller. Nanotub. Carbon Nanostructures.,2011, 19, 251-261. doi:10.1080/15363831003721757.
- Monajjemi, M. Liquid-phase exfoliation (LPE) of graphite towards graphene: An ab initio study. Journal of Molecular Liquids 2017, 230, 461-472. doi:10.1016/j.molliq.2017.01.044.
- Tahan, A.; Mollaamin, F.; Monajjemi, M. Thermochemistry and NBO analysis of peptide bond: Investigation of basis sets and binding energy. Russian Journal of Physical Chemistry A 2009, 83, 587-597. doi:10.1134/S003602440904013X.
- Monajjemi, M.; Baheri, H.; Mollaamin, F. A percolation model for carbon nanotube-polymer composites using the Mandelbrot-Given. Journal of Structural Chemistry 2011, 52, 54-59. doi: 10.1134/S0022476611010070.
- Mahdavian, L.; Monajjemi, M. Alcohol sensors based on SWNT as chemical sensors: Monte Carlo and Langevin dynamics simulation. Microelectronics journal 2010, 41, 142-149. doi: 10.1016/j.mejo.2010.01.011.
- Monajjemi, M. Cell membrane causes the lipid bilayers to behave as variable capacitors: A resonance with self-induction of helical proteins. Biophysical Chemistry 2015, 207, 114-127.
- doi:10.1016/j.bpc.2015.10.003.
- Frisch, M.J.; Trucks, G.W.; Schlegel, H.B.; Scuseria, G.E.; Robb, M.A.; Cheeseman, J.R.; Scalmani, G.; et al. Gaussian, Inc., Wallingford CT. 2009.
- (a) Lee, C.; Yang, W.; Parr, R.G. Development of the Colle-Salvetti Correlation-Energy Formula into a Functional of the Electron Density. Phys. Rev. B, 1988, 37, 785-789. doi:10.1103/PhysRevB.37.785. (b) Stephens, P.J.; Devlin, F.J.; Chabalowski, C.F.; Frisch, M.J. Ab Initio Calculation of Vibrational Absorption and Circular Dichroism Spectra Using Density Functional Force Fields. J. Phys. Chem.,1994, 9 , 11623-11627. doi:10.1021/j100096a001.
- Koch, W.; Holthausen, M.C. A Chemist’s Guide to Density Functional Theory. 3-64, 93-104, 2nd edition, Wiley-VCH, Weinheim, Federal Republic of Germany, 2000.
- (a) Becke, A.D. Density-Functional Thermochemistry. III. The Role of Exact Exchange. J. Chem. Phys. 1993, 98, 5648-5652. doi:10.1063/1.464913. (b) Becke, A.D. Density-Functional Exchange-Energy Approximation with Correct Asymptotic Behavior. Phys. Rev. A. 1988, 38, 3098-3100. doi: 10.1103/PhysRevA.38.3098.
- Bakhshi, K.; Mollaamin, F.; Monajjemi, M. Exchange and correlation effect of hydrogen chemisorption on nano V(100) surface: A DFT study by generalized gradient approximation (GGA). J.Comput.Theor.Nanosci, 2011, 8,763-768. doi: 10.1166/jctn.2011.1750.
- Monajjemi, M.; Najafpour, J.; Mollaamin, F. (3,3)4 Armchair carbon nanotube in connection with PNP and NPN junctions: Ab Initio and DFT-based studies. Fullerenes Nanotubes and Carbon Nanostructures 2013, 21, 213-232. doi:10.1080/1536383X.2011.597010.
- Cramer, C.J.; Truhlar, D.G. PM3-SM3: A general parameterization for including aqueous solvation effects in the PM3 molecular orbital model. J.Comp.Chem. 1992, 13, 1089-1097.doi:10.1002/jcc.540130907.
- Liotard, D.A.; Hawkins, G.D.; Lynch, G.C.; Cramer, C.J.; Truhlar, D.G. Improved methods for semiempirical solvation models. J.Comp.Chem.1995, 16, 422-440. doi: 10.1002/jcc.540160405.
- Mollaamin, F., Shahriari, S.; Monajjemi, M. Drug design of medicinal plants as a treatment of Omicron variant (COVID-19 VARIANT B.1.1.529) , J. Chil. Chem. Soc. 2022, 67, 5562-5570.
- Giesen, D.J.; Gu, M.Z.; Cramer, C.J.; Truhlar, D.G. A Universal Organic Solvation Model. J.Org.Chem. 1996, 61, 8720-8721. doi:10.1021/jo9617427.
- Onsager, L.J. Electric Moments of Molecules in Liquids. J. Am. Chem. Soc. 1936, 58, 1486-1493. doi: 10.1021/ja01299a050.
- Tomasi, J. Cavity and reaction field: “robust” concepts. Perspective on Electric moments of molecules in liquids. Theor.Chem.Acc. 2000, 103, 196-199. doi: 10.1007/s002149900044.
- Sarasia, E.M.; Afsharnezhad, S.; Honarparvar, B.; Mollaamin, F.; Monajjemi, M. Theoretical study of solvent effect on NMR shielding tensors of luciferin derivatives. Phys Chem Liquids 2011, 49, 561-571. doi: 10.1080/00319101003698992.
- Mollaamin, F.; Monajjemi, M.; Salemi, S.; Baei, M.T. A Dielectric Effect on Normal Mode Analysis and Symmetry of BNNT Nanotube. Fuller. Nanotub. Carbon Nanostructures 2011, 19, 182-196. doi: 10.1080/15363831003782932.
- Monajjemi, M.; Robert, W.J.; Boggs, J.E. NMR contour maps as a new parameter of carboxyl's OH groups in amino acids recognition: A reason of tRNA-amino acid conjugation. Chemical Physics 2014, 433, 1-11. doi: 10.1016/j.chemphys.2014.01.017.
- Fry,R.A.; Kwon,K.D.; Komarneni, S.; Kubicki, J.D.; Mueller,K.T. Solid-State NMR and Computational Chemistry Study of Mononucleotides Adsorbed to Alumina, Langmuir, 2006, 22, 9281-9286. doi: 10.1021/la061561s.
- Monajjemi, M.; Mahdavian, L.; Mollaamin, F.; Khaleghian, M. Interaction of Na, Mg, Al, Si with carbon nanotube (CNT): NMR and IR study. Russ. J. Inorg. Chem 2009, 54, 1465-1473. doi: 10.1134/S0036023609090216.
- Aihara, J.; Reduced HOMO−LUMO Gap as an Index of Kinetic Stability for Polycyclic Aromatic Hydrocarbons. J. Phys. Chem. A 1999, 103, 37, 7487-7495. doi: 10.1021/jp990092i.
- Kohn, W.; Becke, A.D.; Parr, R.G. Density Functional Theory of Electronic Structure. J. Phys. Chem. 1996, 100, 12974-12980. doi: 10.1021/jp960669l.
- Parr, R.G. and Pearson, R.G. (1983) Absolute Hardness: Companion Parameter to Absolute Electronegativity. J. Am. Chem. Soc. 1983, 105, 7512-7516. doi: 10.1021/ja00364a005.
- Politzer, P.; Abu-Awwad, F. A comparative analysis of Hartree-Fock and Kohn-Sham orbital energies. Theor. Chem. Acc. 1998, 99, 83-87. doi: 10.1007/s002140050307.
- Silverstein, R.M.; Bassler, G.C.; Morrill, T.C. Spectrometric Identification of Organic Compounds, 5th ed., John Wiley & Sons, Inc., New York, 1981.