MOLECULAR DYNAMIC AND DOCKING STUDY OF CHEMICAL STRUCTURE OF NEW CORONA VIRUSES LINEAGES OF OMICRON BA.n SUB-VARIANTS (n=1-5); BA.4 OR BA.5 STRAINS EXHIBIT THE MOST CONCERN
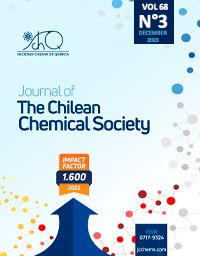
- Omicron,
- BA.4,
- BA.5,
- docking,
- Covid-19
Copyright (c) 2023 SChQ

This work is licensed under a Creative Commons Attribution-NonCommercial-ShareAlike 4.0 International License.
Abstract
The first dominant of Omicron-Covid-19 (BA.1) was produced around thirty mutations in its Spike protein in 2019. Quickly BA.1 became the dominant variant worldwide. Omicron is dangerous for public health concern due to its high infectivity and antibody evasion. Omicron has three lineages or sub variants, BA.1, BA.2, and BA.3. Among them, BA.1 is the currently prevailing sub variant. Omicron BA.1 has around 65 mutations on non-structure protein (NSP3), NSP4, NSP5, NSP6, NSP12, NSP14, S protein, envelope protein, membrane protein, and nucleus capsid proteins. BA.4 and BA.5 are two newly-designated Omicron lineages. They are Omicron viruses with a new combination of mutations containing critical spike protein as a concern for human. In terms of their mutations, BA.4 and BA.5 share mutations across their genomes with both BA.1 and BA.2, but are most similar to BA.2. L452R that previously seen in Kappa, Delta, Epsilon variants and also F486V, and R493 can be seen in both BA.4 and BA.5 where differ from one another in mutations that are outside of the spike gene Data on BA.4 and BA.5, which were first detected in South Africa in early 2022, remain limited. But, these variants seem to spread more quickly than earlier versions of Omicron, such as BA.2, and may be better at dodging the immune system’s defenses. By this work, we simulated the spike protein structures, along with peptide-like inhibitor structure of the 7QO7, 7WE9, 7WPC and 7DF4 structures including small-molecule inhibitors, via molecular dynamic and docking methods. Several genomes of various coronaviruses using BAST and MAFFT software have been evaluated.
References
- Woo, P.C.Y.; Lau, S.K.P.; Lam, C.S.F.; Lau, C.C.Y.; Tsang, A.K.L.; Lau, J.H.N.; Bai, R.; Teng, J.L.L.; Tsang, C.C.C.; Wang, M.; Zheng, B.-J.; Chan, K.-H.; Yuen, K.-Y. Discovery of seven novel Mammalian and avian coronaviruses in the genus deltacoronavirus supports bat coronaviruses as the gene source of alphacoronavirus and betacoronavirus and avian coronaviruses as the gene source of gammacoronavirus and deltacoronavirus. Journal of virology 2012, 86, 3995-4008, https://doi.org/10.1128/JVI.06540-11.
- Zhang, J. Porcine deltacoronavirus: Overview of infection dynamics, diagnostic methods, prevalence and genetic evolution. Virus Res. 2016, 226, 71–84, https://doi.org/10.1016/j.virusres.2016.05.028.
- Wille, M.; Holmes, E.C. Wild birds as reservoirs for diverse and abundant gamma- and deltacoronaviruses. FEMS Microbiol. Rev. 2020, 44, 631–644, https://doi.org/10.1093/femsre/fuaa026.
- Jung, K.; Hu, H.; Saif, L.J. Calves are susceptible to infection with the newly emerged porcine deltacoronavirus, but not with the swine enteric alphacoronavirus, porcine epidemic diarrhea virus. Arch. Virol. 2017, 162, 2357–2362, https://doi.org/10.1007/s00705-017-3351-z.
- Liang, Q.; Zhang, H.; Li, B.; Ding, Q.; Wang, Y.; Gao, W.; Guo, D.; Wei, Z.; Hu, H. Susceptibility of Chickens to Porcine Deltacoronavirus Infection. Viruses 2019, 11, https://doi.org/10.3390/v11060573.
- Boley, P.A.; Alhamo, M.A.; Lossie, G.; Yadav, K.K.; Vasquez-Lee, M.; Saif, L.J.; Kenney, S.P.; Boley, P. Porcine Deltacoronavirus Infection and Transmission in Poultry, United States1. Emerg. Infect. Dis. 2020, 26, 255–265, https://doi.org/10.3201/eid2602.190346.
- Fang, P.; Fang, L.; Hong, Y.; Liu, X.; Dong, N.; Ma, P.; Bi, J.; Wang, D.; Xiao, S. Discovery of a novel accessory protein NS7a encoded by porcine deltacoronavirus. J. Gen. Virol. 2017, 98, 173–178, https://doi.org/10.1099/jgv.0.000690.
- Fang, P.; Fang, L.; Liu, X.; Hong, Y.; Wang, Y.; Dong, N.; Ma, P.; Bi, J.; Wang, D.; Xiao, S. Identification and subcellular localization of porcine deltacoronavirus accessory protein NS6. Virology 2016, 499, 170–177, https://dx.doi.org/10.1016%2Fj.virol.2016.09.015.
- Luo, J.; Fang, L.; Dong, N.; Fang, P.; . Ding, Z.; Wang, D.; Chen, H.; Xiao, S. Porcine deltacoronavirus (PDCoV) infection suppresses RIG-I-mediated interferon-beta production. Virology 2016, 495, 10–17, https://doi.org/10.1016/j.virol.2016.04.025.
- Fang, P.; Fang, L.; Ren, J.; Hong, Y.; Liu, X.; Zhao, Y.; Wang, D.; Peng, G.; Xiao, S. Porcine Deltacoronavirus Accessory Protein NS6 Antagonizes Interferon Beta Production by Interfering with the Binding of RIG-I/MDA5 to Double-Stranded RNA. J. Virol. 2018, 92, e00712-18, https://doi.org/10.1128/jvi.00712-18.
- Fang, P.; Fang, L.; Xia, S.; Ren, J.; Zhang, J.; Bai, D.; Zhou, Y.; Peng, G.; Zhao, S.; Xiao, S. Porcine Deltacoronavirus Accessory Protein NS7a Antagonizes IFN-beta Production by Competing With TRAF3 and IRF3 for Binding to IKKepsilon. Front. Cell. Infect. Microbiol. 2020, 10, https://dx.doi.org /10.3389%2Ffcimb.2020.00257
- Haraway, D. A partilha do sofrimento: relações instrumentais entre animais de laboratório e sua gente. Horizontes Antropo lógicos 2011, 17, 27-64, https://doi.org/10.1590/S0104-71832011000100002.
- Jung, K.; Miyazaki, A.; Hu, H.; Saif, L.J. Susceptibility of porcine IPEC-J2 intestinal epithelial cells to infection with porcine deltacoronavirus (PDCoV) and serum cytokine responses of gnotobiotic pigs to acute infection with IPEC-J2 cell culture-passaged PDCoV. Vet. Microbiol. 2018, 221, 49–58, https://doi.org/10.1016/j.vetmic.2018.05.019.
- Lee, Y.J.; Lee, C. Porcine deltacoronavirus induces caspase-dependent apoptosis through activation of the cytochrome c –mediated intrinsic mitochondrial pathway. Virus Res. 2018, 253, 112–123, https://doi.org/10.1016/j.virusres.2018.06.008.
- Jung, K.; Hu, H.; Saif, L.J. Porcine deltacoronavirus induces apoptosis in swine testicular and LLC porcine kidney cell lines in vitro but not in infected intestinal enterocytes in vivo. Vet. Microbiol. 2016, 182, 57–63, https://doi.org/10.1016/j.vetmic.2015.10.022.
- Keck, F. Avian reservoirs: virus hunters and birdwatchers in Chinese sentinel posts. Duke University Press 2020, https://doi.org/10.1215/9781478007555.
- Keck, F.; Lynteris, C. Zoonosis: prospects and challenges for medical anthropology. Medicine Anthropology Theory 2018, 5, https://doi.org/10.17157/mat.5.3.372.
- Paxson, H. Post-Pasteurian cultures: the microbiopolitics of raw-milk cheese in the United States. Cultural Anthropology 2008, 23, 15–47, https://doi.org/10.1111/j.1548-1360.2008.00002.x.
- Segata, J.; Mastrangelo, A. As biosseguranças e suas antropologias. Horizontes Antropológicos 2020, 26, 7-25, https://doi.org/10.1590/S0104-71832020000200001.
- Singer, M.; Rylko-Bauer, B. The syndemics and structural violence of the Covid pandemic: anthropological insights on a crisis. Open Anthropology Research 2021, 1, 7-32, https://doi.org/10.1515/opan-2020-0100.
- Kirksey, E.; Helmreich, S. The emergence of multispecies ethnography. Cultural Anthropology 2010, 25, 545-576, https://doi.org/10.1111/j.1548-1360.2010.01069.x.
- Law, J. Care and killing: tensions in veterinary practice. In: Mol, A.; Moser, I.; Pols, J. (Eds). Care in practice: on tinkering in clinics, homes and farms. Transcript 2015, 57-72, Bielefeld, https://doi.org/10.1515/transcript.9783839414477.57.
- Lodder, W.; Husman, A. SARS-CoV-2 in wastewater: potential health risk, but also data source. Lancet Gatroenterol Hepatol. 2020, 5, 533-534, https://doi.org/10.1016/s2468-1253(20)30087-x.
- Nading, A. Human, animals and health: from ecology to entanglement. Environment and Society: Advances in Research 2013, 4, 60-78, https://doi.org/10.3167/ares.2013.040105.
- Caduff, C. On the verge of death: visions of biological vulnerability. Annual Review of Anthropology 2014, 43, 105-121, https://doi.org/10.1146/annurev-anthro-102313-030341.
- Hall, V.J.; Foulkes, S.; Charlett, A. et al. SARS-CoV-2 infection rates of antibody-positive compared with antibody-negative healthcare workers in England: a large, multicentre, prospective cohort study (SIREN). The Lancet 2021, 397, 1459-1469, https://doi.org/10.1016/s0140-6736(21)00675-9.
- Hansen, C.H.; Michlmayr, D.; Gubbels, S.M.; Mølbak, K.; Ethelberg, S. Assessment of protection against reinfection with SARS-CoV-2 among 4 million PCR-tested individuals in Denmark in 2020: a population-level observational study. The Lancet 2021, 397, 1204-1212, https://doi.org/10.1016/S0140-6736(21)00575-4.
- Cele, S.; Gazy, I.; Jackson, L. et al. Escape of SARS-CoV-2 501Y.V2 from neutralization by convalescent plasma. Nature 2021, 593, 142-146, https://doi.org/10.1038/s41586-021-03471-w.
- Wibmer, C.K.; Ayres, F.; Hermanus, T. et al. SARS-CoV-2 501Y.V2 escapes neutralization by South African COVID- 19 donor plasma. Nat Med. 2021, 7, 622-625, https://doi.org/10.1038/s41591-021-01285-x.
- Planas, D.; Veyer, D.; Baidaliuk, A. et al. Reduced sensitivity of SARS-CoV-2 variant Delta to antibody neutralization. Nature 2021, 596, 276-280, https://doi.org/10.1038/s41586-021-03777-9.
- Liu, C.; Ginn, H.M.; Dejnirattisai, W. et al. Reduced neutralization of SARS-CoV-2 B.1.617 by vaccine and convalescent serum. Cell. 2021, 184, 4220-4236.e13, https://doi.org/10.1016/j.cell.2021.06.020.
- Tegally, H.; Wilkinson, E.; Giovanetti, M. et al. Detection of a SARS-CoV-2 variant of concern in South Africa. Nature 2021, 592, 438-443, https://doi.org/10.1038/s41586-021-03402-9.
- Shu, Y.; McCauley, J. GISAID: Global initiative on sharing all influenza data – from vision to reality. Euro Surveillance 2017, 22, 30494, https://dx.doi.org/10.2807%2F1560-7917.ES.2017.22.13.30494.
- Murchu, E.O.; Byrne, P.; Carty, P.G. et al. Quantifying the risk of SARS-CoV-2 reinfection over time. Rev Med Virol 2021, 32, e2260, https://doi.org/10.1002/rmv.2260.
- Cohen, C.; Kleynhans, J.; von Gottberg, A. et al. SARS-CoV-2 incidence, transmission and reinfection in a rural and an urban setting: results of the PHIRST-C cohort study, South Africa, 2020-2021. medRxiv 2021, https://doi.org/10.1101/2021.07.20.21260855 (Preprint).
- Wang, P.; Nair, M.S.; Liu, L. et al. Antibody resistance of SARS-CoV-2 variants B.1.351 and B.1.1.7. Nature 2021, 593, 130-135, https://doi.org/10.1038/s41586-021-03398-2.
- Cho, A.; Muecksch, F.; Schaefer-Babajew, D. et al. Anti-SARS-CoV-2 receptor-binding domain antibody evolution after mRNA vaccination. Nature 2021, 600, 517-522, https://doi.org/10.1038/s41586-021-04060-7.
- Monajjemi, M. Cell membrane causes the lipid bilayers to behave as variable capacitors: A resonance with self-induction of helical proteins. Biophysical Chemistry 2015, 207, 114-127, https://doi.org/10.1016/j.bpc.2015.10.003.
- Ghalandari, B.; Monajjemi, M.; Mollaamin, F. Theoretical Investigation of Carbon Nanotube Binding to DNA in View of Drug Delivery. J.Comput.Theor.Nanosci 2011, 8, 1212-1219, https://doi.org/10.1166/jctn.2011.1801.
- Monajjemi, M. Liquid-phase exfoliation (LPE) of graphite towards graphene: An ab initio study. Journal of Molecular Liquids 2017, 230, 461–472, https://doi.org/10.1016/j.molliq.2017.01.044.
- Tahan, A.; Mollaamin, F.; Monajjemi, M. Thermochemistry and NBO analysis of peptide bond: Investigation of basis sets and binding energy. Russian Journal of Physical Chemistry A 2009, 83, 587-597, https://doi.org/10.1134/S003602440904013X.
- Monajjemi, M.; Robert, W.J.; Boggs, J.E. NMR contour maps as a new parameter of carboxyl's OH groups in amino acids recognition: A reason of tRNA–amino acid conjugation. Chemical Physics 2014, 433, 1-11, https://doi.org/10.1016/j.chemphys.2014.01.017.
- Sarasia, E.M.; Afsharnezhad, S.; Honarparvar, B.; Mollaamin, F.; Monajjemi, M. Theoretical study of solvent effect on NMR shielding tensors of luciferin derivatives. Phys Chem Liquids 2011, 49, 561-571, https://doi.org/10.1080/00319101003698992.
- Monajjemi, M.; Heshmat, M.; Haeri, H.H.; Kaveh, F. Theoretical study of vitamin properties from combined QM-MM methods: Comparison of chemical shifts and energy. Russian Journal of Physical Chemistry 2006, 80, 1061, https://doi.org/10.1134/S0036024406070119.
- Khaleghian, M.; Zahmatkesh, M.; Mollaamin, F.; Monajjemi, M. Investigation of Solvent Effects on Armchair Single-Walled Carbon Nanotubes: A QM/MD Study. Fuller. Nanotub. Carbon Nanostructures.,2011, 19, 251-261, https://doi.org/10.1080/15363831003721757.
- Monajjemi, M.; Honaparvar, B.; Khalili Hadad, B.; Ilkhani, A.; Mollaamin, F. Thermo-Chemical
- Investigation and NBO Analysis of Some anxileotic as Nano- Drugs. African journal of pharmacy and pharmacology 2010, 4, 521-529.
- Jiahui Chen, Rui Wang, Nancy Benovich Gilby, and Guo-Wei Wei. Omicron variant (b. 1.1. 529): Infectivity, vaccine breakthrough, and antibody resistance. Journal of chemical information and modeling,
- Huiping Shuai, Jasper Fuk-Woo Chan, Bingjie Hu, Yue Chai, Terrence Tsz-Tai Yuen, Feifei Yin, Xiner
- Huang, Chaemin Yoon, Jing-Chu Hu, Huan Liu, et al. Attenuated replication and pathogenicity of
- sars-cov-2 b. 1.1. 529 omicron. Nature, pages 1-2 , 2022.
- Sandile Cele, Laurelle Jackson, David S Khoury, Khadija Khan, Thandeka Moyo-Gwete, Houriiyah
- Tegally, James Emmanuel San, Deborah Cromer, Cathrine Scheepers, Daniel G Amoako, et al. Omicron
- extensively but incompletely escapes pfizer bnt162b2 neutralization. Nature, pages 1-5, 2021.
- Li Zhang, Qianqian Li, Ziteng Liang, Tao Li, Shuo Liu, Qianqian Cui, Jianhui Nie, Qian Wu, Xiaowang
- Qu, Weijin Huang, et al. The significant immune escape of pseudotyped sars-cov-2 variant omicron.
- Emerging microbes & infections, 11(1), pages; 1-5, 2022.
- Lihong Liu, Sho Iketani, Yicheng Guo, Jasper FW Chan, Maple Wang, Liyuan Liu, Yang Luo, Hin
- Chu, Yiming Huang, Manoj S Nair, et al. Striking antibody evasion manifested by the omicron variant of sars- cov-2. Nature, pages 1-8, 2021.
- Markus Hoffmann, Hannah Kleine-Weber, Simon Schroeder, Nadine Kr¨uger, Tanja Herrler, Sandra
- Erichsen, Tobias S Schiergens, Georg Herrler, Nai-Huei Wu, Andreas Nitsche, et al. SARS-CoV-2 cell
- entry depends on ACE2 and TMPRSS2 and is blocked by a clinically proven protease inhibitor. Cell,
- (2):271-280, 2020.
- Jiahui Chen, Rui Wang, Menglun Wang, and Guo-Wei Wei. Mutations strengthened SARS-CoV-2
- infectivity. Journal of molecular biology, 432(19), pages; 5212-5226, 2020.
- Rui Wang, Jiahui Chen, Kaifu Gao, and Guo-Wei Wei. Vaccine-escape and fast-growing mutations
- in the United Kingdom, the United States, Singapore, Spain, India, and other COVID-19-devastated
- countries. Genomics, 113(4), pages; 2158-2170, 2021.
- Chunyan Wang, Wentao Li, Dubravka Drabek, Nisreen MA Okba, Rien van Haperen, Albert DME
- Osterhaus, Frank JM van Kuppeveld, Bart L Haagmans, Frank Grosveld, and Berend-Jan Bosch. A
- human monoclonal antibody blocking SARS-CoV-2 infection. Nature communications, 11(1), pages:1-6, 2020.
- Fei Yu, Rong Xiang, Xiaoqian Deng, Lili Wang, Zhengsen Yu, Shijun Tian, Ruiying Liang, Yanbai
- Li, Tianlei Ying, and Shibo Jiang. Receptor-binding domain-specific human neutralizing monoclonal
- antibodies against SARS-CoV and SARS-CoV-2. Signal Transduction and Targeted Therapy, 5(1), pages1-12,
- Cheng Li, Xiaolong Tian, Xiaodong Jia, Jinkai Wan, Lu Lu, Shibo Jiang, Fei Lan, Yinying Lu, Yanling Wu, and Tianlei Ying. The impact of receptor-binding domain natural mutations on antibody recognition of SARS-CoV-2. Signal Transduction and Targeted Therapy, 6(1), pages: 1-3, 2021.
- Jiahui Chen, Kaifu Gao, Rui Wang, and Guo-Wei Wei. Prediction and mitigation of mutation threats to COVID -19 vaccines and antibody therapies. Chemical Science, 12(20), pages: 6929-6948, 2021.
- Bryan E Jones, Patricia L Brown-Augsburger, Kizzmekia S Corbett, Kathryn Westendorf, Julian Davies, Thomas P Cujec, Christopher M Wiethoff, Jamie L Blackbourne, Beverly A Heinz, Denisa Foster, et al. The neutralizing antibody, ly-cov555, protects against sars-cov-2 infection in nonhuman primates. Science translational medicine, 13,593 ), 2021.
- Rui Shi, Chao Shan, Xiaomin Duan, Zhihai Chen, Peipei Liu, Jinwen Song, Tao Song, Xiaoshan Bi, Chao Han, Lianao Wu, et al. A human neutralizing antibody targets the receptor binding site of SARS- CoV -2. Nature, pages 1-8, 2020.
- Johanna Hansen, Alina Baum, Kristen E Pascal, Vincenzo Russo, Stephanie Giordano, Elzbieta Wloga, Benjamin O Fulton, Ying Yan, Katrina Koon, Krunal Patel, et al. Studies in humanized mice and convalescent humans yield a SARS-CoV-2 antibody cocktail. Science, 369(6506):1010-1014, 2020.
- Jinhui Dong, Seth J Zost, Allison J Greaney, Tyler N Starr, Adam S Dingens, Elaine C Chen, Rita E Chen, James Brett Case, Rachel E Sutton, Pavlo Gilchuk, et al. Genetic and structural basis for sars-cov-2 variant neutralization by a two-antibody cocktail. Nature Microbiology, 6(10):1233{1244, 2021.
- Zixuan Cang and Guo-Wei Wei, Analysis and prediction of protein folding energy changes upon mutation
- by element specific persistent homology. Bioinformatics, 33(22):3549{3557, 2017.
- Jiahui Chen, Kaifu Gao, Rui Wang, and Guo-Wei Wei. Revealing the threat of emerging SARS-CoV-2
- mutations to antibody therapies. Journal of Molecular Biology, 433(7744), 2021
- Justina Jankauskait_e, Brian Jim´enez-Garc´ıa, Justas Dapk¯unas, Juan Fern´andez-Recio, and Iain H Moal.
- SKEMPI 2.0: an updated benchmark of changes in protein{protein binding energy, kinetics and thermo dynamics upon mutation. Bioinformatics, 35(3):462-469, 2019.
- Kui K Chan, Danielle Dorosky, Preeti Sharma, Shawn A Abbasi, John M Dye, David M Kranz, Andrew S
- Herbert, and Erik Procko. Engineering human ACE2 to optimize binding to the spike protein of SARS
- coronavirus 2. Science, 369(6508):1261{1265, 2020.
- Tyler N Starr, Allison J Greaney, Sarah K Hilton, Daniel Ellis, Katharine HD Crawford, Adam S Dingens, Mary Jane Navarro, John E Bowen, M Alejandra Tortorici, Alexandra C Walls, et al. Deep mutational scanning of SARS-CoV-2 receptor binding domain reveals constraints on folding and ACE2 binding. Cell, 182(5):1295-1310, 2020.
- Thomas W Linsky, Renan Vergara, Nuria Codina, Jorgen W Nelson, Matthew J Walker, Wen Su, Christopher O Barnes, Tien-Ying Hsiang, Katharina Esser-Nobis, Kevin Yu, et al. De novo design of potent and resilient hACE2 decoys to neutralize SARS-CoV-2. Science, 370(6521):1208{1214, 2020.
- Menglun Wang, Zixuan Cang, and Guo-Wei Wei. A topology-based network tree for the prediction of protein binding affinity changes following mutation. Nature Machine Intelligence, 2(2):116-123, 2020.
- E. Callaway, New Omicron relatives BA.4 and BA.5 offer hints about the future of SARS-CoV-2, Nature 605 (2022) 204–206.
- R. Khandia, S. Singhal, T. Alqahtani, M.A. Kamal, N.A. El-Shall, F. Nainu, P. A. Desingu, K. Dhama, Emergence of SARS-CoV-2 Omicron (B.1.1.529) variant, salient features, high global health concerns and strategies to counter it amid ongoing COVID-19 pandemic, Environ. Res. 209 (2022), 112816, https://doi.org/10.1016/j.envres.2022.112816.
- R.K. Mohapatra, V. Kandi, S. Verma, K. Dhama, Challenges of the Omicron (B.1.1.529) variant and its lineages: a global perspective, Chembiochem 23 (9) (2022), https://doi.org/10.1002/cbic.202200059.
- D. Yamasoba, Y. Kosugi, I. Kimura, S. Fujita, K. Uriu, J. Ito, K. Sato, Sensitivity of novel SARS-CoV-2 Omicron subvariants, BA.2.11, BA.2.12.1, BA.4 and BA.5 to therapeutic monoclonal antibodies [preprint] May 3, bioRxiv (2022), https://doi. org/10.1101/2022.05.03.490409.
- H. Tegally, M. Moir, J. Everatt, M. Giovanetti, C. Scheepers, E. Wilkinson, K. Subramoney, S. Moyo, D.G. Amoako, C. Baxter, C.L. Althaus, U.J. Anyaneji, D. Kekana, R. Viana, J. Giandhari, R.J. Lessells, T. Maponga, D. Maruapula, W. Choga, M. Matshaba, S. Mayaphi, N. Mbhele, M.B. Mbulawa, N. Msomi, N.G.S.- S.A. consortium, Y. Naidoo, S. Pillay, T.J. Sanko, J.E. San, L. Scott, L. Singh, N. A. Magini, P. Smith-Lawrence, W. Stevens, G. Dor, D. Tshiabuila, N. Wolter, W. Preiser, F.K. Treurnicht, M. Venter, M. Davids, G. Chiloane, A. Mendes, C. McIntyre, A. O’Toole, C. Ruis, T.P. Peacock, C. Roemer, C. Williamson, O. G. Pybus, J. Bhiman, A. Glass, D.P. Martin, A. Rambaut, S. Gaseitsiwe, A. von Gottberg, T. de Oliveira, Continued emergence and evolution of Omicron in South Africa: new BA.4 and BA.5 lineages [preprint] May 2, medRxiv (2022), https://doi. org/10.1101/2022.05.01.22274406.
- ECDC, Epidemiological Update: SARS-CoV-2 Omicron Sub-lineages BA.4 and BA.5, 2022, 17-05-2022.
- WHO, Tracking SARS-CoV-2 variants. https://www.who.int/activities/trackingSARS-CoV-2-variants, 2022. (Accessed 17 May 2022).
- X. Xie, Y. Cao, A. Yisimayi, F. Jian, et al., [Preprint] ResearchSquare, 2022, https://doi.org/10.21203/rs.3.rs-1611421/v1, 2022.
- Gobeil, S.M., Janowska, K., McDowell, S., Mansouri, K., Parks, R., Stalls, V., Kopp, M.F., Manne, K., Li, D., Wiehe, K., et al. (2021). Effect of natural mutations of SARS-CoV-2 on spike structure, conformation, and antigenicity. Science 373, 6555
- Clark, S.A., Clark, L.E., Pan, J., Coscia, A., McKay, L.G.A., Shankar, S., Johnson, R.I., Brusic, V., Choudhary, M.C., Regan, J., et al. (2021). SARS-CoV-2 evolution in an immune compromised host reveals shared neutralization escape mechanisms. Cell 184, 2605–2617. e18.
- Nutalai, R., Zhou, D., Tuekprakhon, A., Ginn, H.M., Supasa, P., Liu, C., Huo, J., Mentzer, A.J., Duyvesteyn, H.M.E., Dijokaite-Guraliuc, A., et al. (2022). Potent cross-reactive antibodies following Omicron breakthrough in vaccinees. Cell. https://doi.org/10.1016/j.cell.2022.05.014.
- Aekkachai Tuekprakhon, Rungtiwa Nutalai, Aiste Dijokaite-Guraliuc, Daming Zhou, Helen M. Ginn, et.al. Antibody escape of SARS-CoV-2 Omicron BA.4 and BA.5 from vaccine and BA.1 serum, Cell 185, 2422–2433, July 7, 2022
- Hachmann NP, Miller J, Collier A ris Y, Ventura JD, Yu J, Rowe M, et al. Neutralization Escape by the SARS-CoV-2 Omicron Variants BA.2.12.1 and BA.4/BA.5 [Internet]. medRxiv; 2022 May [cited 2022 2022 May 31] p. 2022.05.16.22275151. Available from: https://www.medrxiv.org/content/10.1101/2022.05.16.22275151v1
- Wang Q, Guo Y, Iketani S, Li Z, Mohri H, Wang M, et al. SARS-CoV-2 Omicron BA.2.12.1, BA.4, and BA.5 subvariants evolved to extend antibody evasion [Internet]. bioRxiv; 2022 May [cited 2022 May 31] p. 2022. 05. 26. 493517. Available from: https://www.biorxiv.org/content/10.1101/2022.05.26.493517v1
- Tuekprakhon A, Huo J, Nutalai R, Dijokaite-Guraliuc A, Zhou D, Ginn HM, et al. Further antibody escape by Omicron BA.4 and BA.5 from vaccine and BA.1 serum [Internet]. bioRxiv; 2022 May [cited 2022 May 31] p. 2022.05.21.492554. Available from: https://www.biorxiv.org/content/10.1101/2022.05.21.492554v1
- Cao Y, Yisimayi A, Jian F, Song W, Xiao T, Wang L, et al. BA.2.12.1, BA.4 and BA.5 escape antibodies elicited by Omicron infection [Internet]. bioRxiv; 2022 May [cited 2022 May 10] p. 2022.04.30.489997. Available from: https://www.biorxiv.org/content/10.1101/2022.04.30.489997v1.
- CDC. COVID Data Tracker [Internet]. Centers for Disease Control and Prevention. 2020 [cited 2022 Jun 2]. Available from: https://covid.cdc.gov/covid-data-tracker
- Wolter, et al. “Early assessment of the clinical severity of the SARS-CoV-2 omicron variant in South Africa: a data linkage study.” The Lancet vol. 399, 10323 (2022): 437-446. doi:10.1016/S0140-6736(22)00017-4
- Madhi, et al. “Population Immunity and Covid-19 Severity with Omicron Variant in South Africa.” The New England Journal of Medicine vol. 386,14 (2022): 1314-1326. doi:10.1056/NEJMoa2119658.
- Kleynhans, et al. “SARS-CoV-2 Seroprevalence after Third Wave of Infections, South Africa.” Emerging Infectious Diseases vol. 28,5 (2022): 1055-1058. doi:10.3201/eid2805.220278.
- Zhang W, Huang L, Ye G, et al. Vaccine booster eÕciently inhibits entry of SARS-CoV-2 omicron variant. Cell Mol Immunol. 2022; 19(3): 445- 446. https://doi.org/10.1038/s41423-022-00837-6.
- Garcia-Beltran WF, StDenis KJ, Hoelzemer A, et al. mRNA-based COVID-19 vaccine boosters induce neutralizing immunity against SARS-CoV-2 Omicron variant. Cell. 2022; 185(3): 457- 466. https://doi.org/10.1016/j.cell.2021.12.033.
- Haveri A, Solastie A, Ekström N, et al. Neutralizing Antibodies to SARS-CoV-2 Omicron Variant after 3rd mRNA Vaccination in Health Care Workers and Elderly Subjects and Response to a Single Dose in Previously Infected Adults; 2021. medRxiv 2021:2021.2012.2022.21268273.
- Nemet I, Kliker L, Lustig Y, et al. Third BNT162b2 vaccination neutralization of SARS-CoV-2
- omicron infection. N Engl J Med. 2021; 386(5): 492- 494. https://doi.org/10.1056/nejmc2119358.
- Gruell H, Vanshylla K, Tober-Lau P, et al. mRNA Booster Immunization Elicits Potent Neutralizing Serum Activity against the SARS-CoV-2 Omicron Variant. medRxiv 2021: 2021. 2012. 2014.21267769.
- Yu X, Wei D, Xu W, et al. Reduced Sensitivity of SARS-CoV-2 Omicron Variant to BoosterEnhanced Neutralization; 2021. medRxiv 2021:2021.2012.2017.21267961.
- Muik A, Lui BG, Wallisch AK, et al. Neutralization of SARS-CoV-2 Omicron by BNT162b2 mRNA vaccine-elicited human sera. Science. 2022; 375(6581): eabn7591- 680.https://doi.org/ 10.1126/science.abn7591.
- Edara V-V, Manning KE, Ellis M, et al. mRNA-1273 and BNT162b2 mRNA vaccines have reduced neutralizing activity against the SARS-CoV-2 omicron variant. Cell Rep Med. 2022; 3(2):100529. https://doi.org/10.1016/j.xcrm.2022.100529.
- Shrestha, L.B.; Foster,C.; Rawlinson,W.; Tedla,N.; Bull. R.A. Evolution of the SARS-CoV-2 omicron variants BA.1 to BA.5: Implications for immune escape and transmission, Rev Med Virol.2022;e2381.1-14. https://doi.org/10.1002/rmv.2381.
- coronaviruses. Nat. Rev. Microbiol. 2019, 17, 181-192, https://doi.org/10.1038/s41579-018-0118-9.
- Park, Y.J.; Walls, A.C.; Wang, Z.; Sauer, M.; Li, W.; Tortorici, M.A.; Bosch, B.J.; DiMaio, F.D.; Veesler, D. Seattle Structural Genomics Center for Infectious Disease (SSGCID). Nat.Struct.Mol.Biol 2019, 26, 1151-1157, https://doi.org/10.1038/s41594-019-0334-7.